QM analyses of Electrophilic Aromatic Iodination
QM Magic Class | Chapter 30
< Magical Power of Quantum Mechanics
Aromatic iodo compounds, with higher reactivity as compared to their bromo and chloro analogs, play an important role in synthetic organic chemistry. In addition to functional group interconversion, iodo intermediates could also be used for preparation of radio labeled analogs. For example, in the preparation of tritium (3H)-labeled HIV-1 integrase inhibitor MK-0248, it is first iodinated, then reduced with sodium borotritide (NaB3H4) for extensive biological evaluations and DMPK profiling (Figure 1).[1]

In chapter 25 we discussed the use of HOMO (nucleophile) and LUMO (electrophile) energy gap to assess how readily they will react with one another, as exemplified with halogenation reagents and a set of substrates for Pictet-Spengler reaction. We also described that phenol readily reacts with N-iodosuccinimide (NIS) to provide a mixture of mono-, di- and tri-iodinated products. However, iodination of electron deficient 4-chloronitrobenzene with NIS proceeds only in the presence of trifluoromethanesulfonic acid. It was reasoned that trifluoromethanesulfonic acid reacts with NIS to generate iodine(I) trifluoromethane-sulfonate,[2] which is the active species for the iodination (Figure 1). At the end of the chapter, we were asked to decide whether we shall use NIS or this super-electrophilic reagent to iodinate 1,2,3-trifluoro-4-nitrobenzene substrate, and predict whether reaction will occur at C-5 or C-6 position (Figure 2).

Regioselectivity and reagent choice are two essential considerations. In this chapter, we will describe how QM analyses could guide us prospectively.
(I) Regioselectivity of iodination reaction
In chapters 2, 17, and 18, we discussed the use of calculated HOMO, HOMO map, and 13C NMR data to predict regioselectivity of NCS chlorination and NBS bromination of various heterocyclic aromatic systems.
These electrophilic reactions usually occur preferentially at substitutable positions with the largest HOMO lobe of the substrates. As such, we first calculate for the frontier orbitals of 1,2,3-trifluoro 4-nitrobenzene and their energies. Since the energy difference between HOMO and HOMO-1 is relatively small, 0.2 eV, we learned from experience to consider both HOMO and HOMO-1 (Figure 3). For HOMO, the lobe is mainly distributed at substitutable C-5, while for HOMO-1, it is mostly on C-6. The differences are not significant enough to enable any confident prediction. Moreover, calculated 13C NMR of the substrate (Figure 3) showed C-5 and C-6 with chemical shift of 123 and 112 ppm, respectively, a difference in 11 ppm, suggesting that electrophilic aromatic reaction will preferentially occur at C-6.
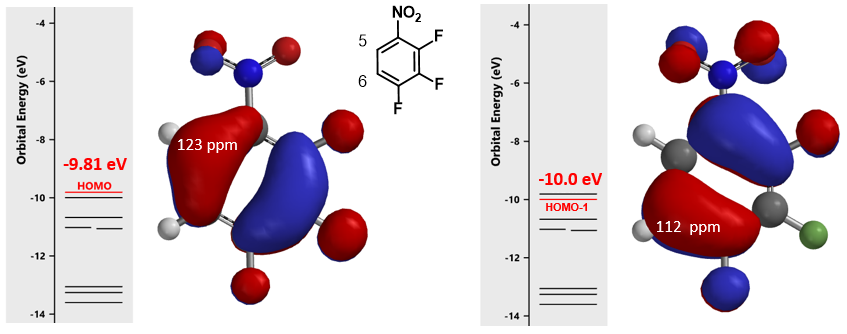
Further comparison of the HOMO and HOMO-1 maps (Figure 4) revealed that the HOMO-1 density at C-6 is significantly higher than that of HOMO at C-5, as indicated by the deeper blue color at C-6 vs C-5, augmenting the prediction from calculated 13C NMR; that is, C-6 position is more likely to undergo selective electrophilic substitution.
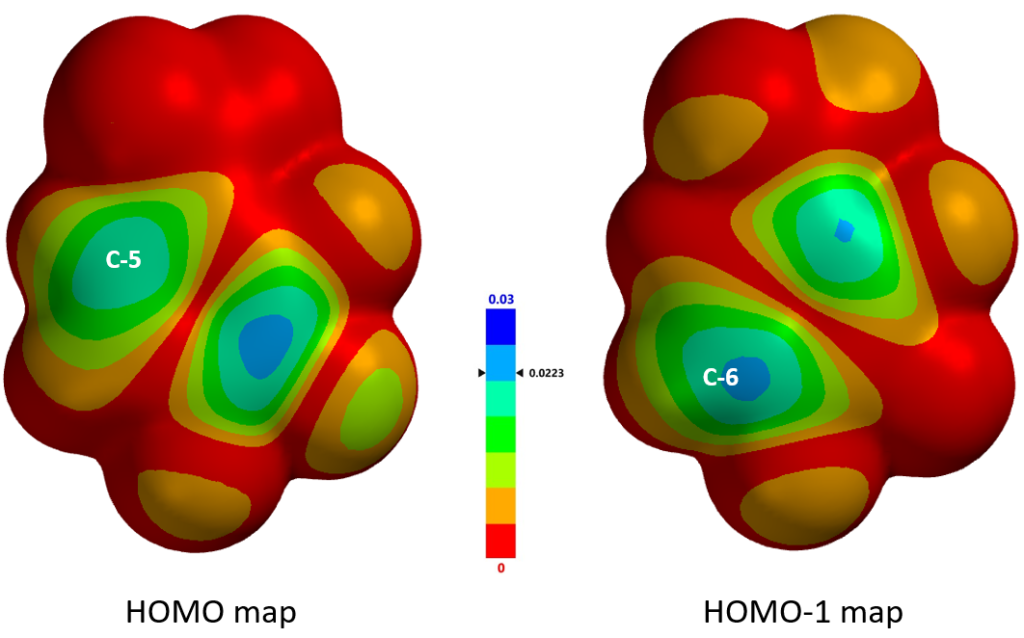
Extending the “Equilibrium Geometry and Relative Energy” comparison method described in chapter 17 to assess regioselectivity in bromination to iodination, the relative energy calculated for equilibrium geometry of C-6 is found to be significantly lower than that of C-5 (Figure 5). We have enough data to make a prediction that the iodination reaction is likely to occur selectively at C-6 position, and this is consistent with experimental result.[2, 3]
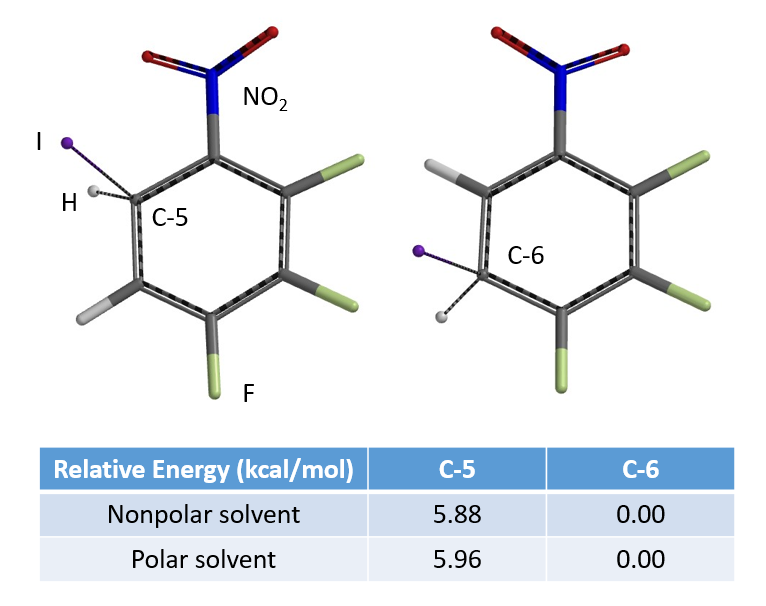
Summarizing key results from the sequence of calculations described above:
- HOMO and HOMO-1 energies differ by only 0.2 eV, requiring both HOMO and HOMO-1 to be considered. HOMO suggests iodination will occur at C-5, whereas HOMO-1 suggests that it will occur at C-6.
- Calculated 13C NMR data predicts that the reaction will occur selectively at C-6.
- HOMO map and HOMO-1 map comparison predicts that iodination will occur selectively at C-6.
- Calculated equilibrium geometry and relative energy of C-5 vs C-6 iodinium ions predict that the reaction will also occur selectively at C-6.
Overall, calculations suggest that the iodination will occur preferentially at C-6, and is consistent with experimental result.[2,3]
(II) Selection of iodination reagent
After determining regioselectivity for iodination of the substrate, we have to select appropriate iodinating reagent (and reaction conditions).
In chapter 25, we discussed that the lower the LUMO energy of a chlorinating reagent, the stronger its reactivity in electrophilic aromatic reaction (Figure 6). For different substrates, their reactivity could be assessed by calculating the energy difference between LUMO of the chlorinating reagent and HOMO of the substrate. When the energy gap exceeds a specific threshold, the reaction will not proceed.
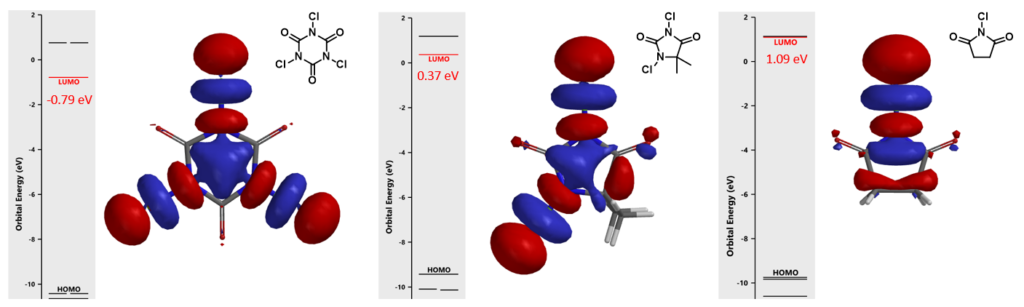
This method is also applicable for assessing the reactivity of the iodination reagents and their reactions.
In iodination of phenol with NIS, the energy difference between LUMO of NIS and HOMO of phenol is 7.52 eV, and the reaction proceeds readily (Table 1). However, under the same conditions, no reaction is observed between 1,2,3-trifluoro-4-nitrobenzene and NIS. The energy gap between HOMO-1 and LUMO of this reacting pair is 9.54 eV, presumably above the threshold. With CF3SO3I as iodinating reagent, the LUMO and substrate HOMO-1 energy gap is lowered to 7.68 eV, and the reaction proceeds smoothly.
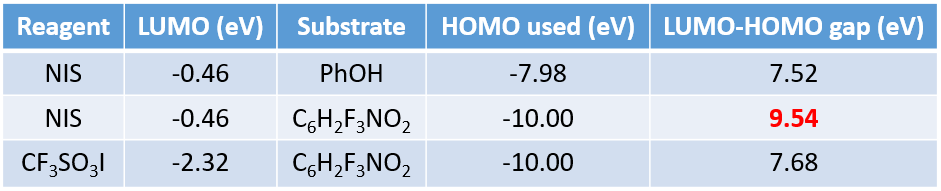
LUMO energies of a few common iodination reagents are calculated and shown on the scale below for comparison (Figure 7). For electron rich substrates, reagents with relatively higher LUMO energy will be sufficient and shall be used to avoid over iodination, whereas for electron deficient substrates, reagents with lower LUMO energy, such as CF3SO3I and CH3SO3I, will be necessary.[4]

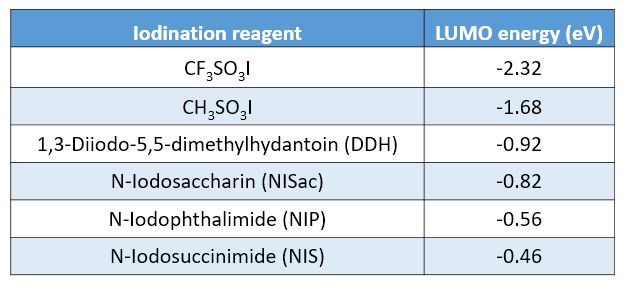
Summary
For electrophilic aromatic iodination, HOMO analysis, calculated 13C NMR data, HOMO map, calculated equilibrium geometries and their relative energy are useful in predicting the site of reaction. Whereas energy difference between LUMO of the iodination reagents and HOMO of the substrates enables assessment of their feasibility. For comparison, a few common iodination reagents are ranked by their LUMO energy to serve as a quick guide to their relative reactivity.
Building on What We Just learned
We need to prepare iodo derivative of the inhibitor below (Figure 8) for tritium labeling. Iodination will occur only on the substitutable carbons with suitable HOMO/HOMO-1 Lobes on the phenyl group. Which carbon will be iodinated and which reagent shall we use?
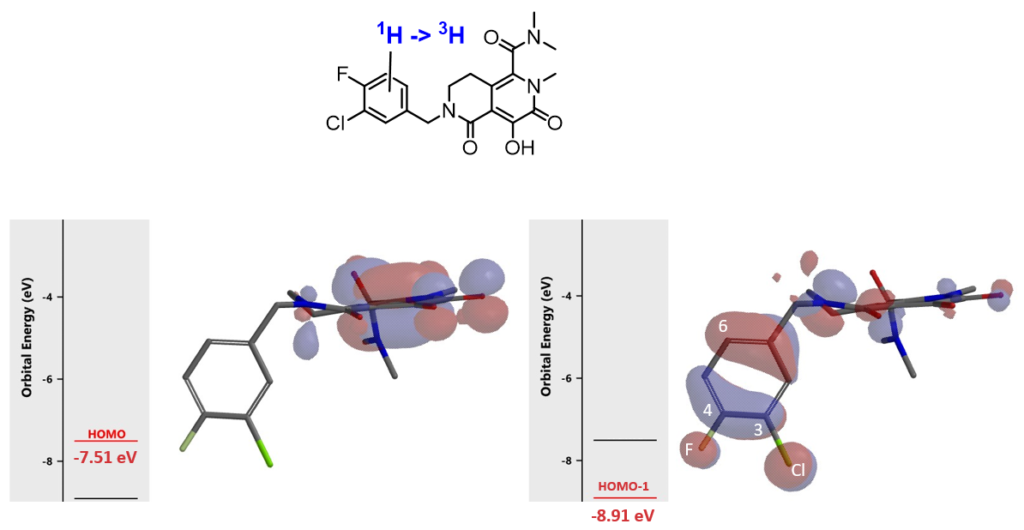
References:
[1] (a) R. Voges, J.R. Heys, T. Moenius, Preparation of Compounds Labeled with Tritium and Carbon-14, John Wiley & Sons Ltd, 2009. (b) T. Nagasaki, K. Sakai, M. Segawa, Y. Katsuyama, N. Haga, M. Koike, K. Kawada, S. Takechi, J. Labelled Cpd. Radiopharm. 2001, 44, 993.
[2] G.A. Olah, Q. Wang, G. Sandford, G.K. Surya Prakash, J. Org. Chem. 1993, 58, 3194.
[3] Spartan’20 QM NMR calculation algorithm is not applicable for iodine containing compounds.
[4] X.S. Xue, Y. Wang, M. Li, J.P. Cheng, J. Org. Chem. 2016, 81, 4280. Fluorinated reagent FPD values (Figure 9).
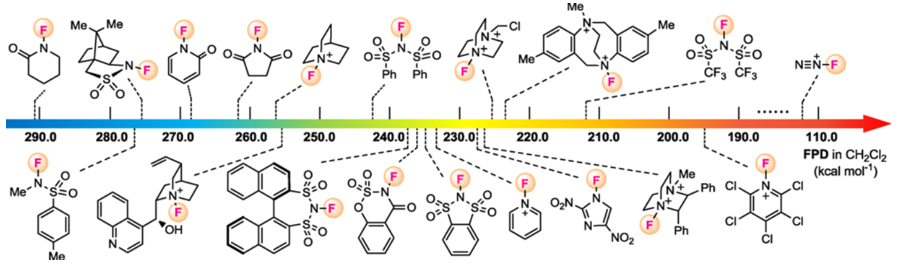
This article is written and edited by Jiahui Lu, Liting Dong, Zhaowu Xu, Qiuyue Wang, Yongsheng Chen, John S. Wai